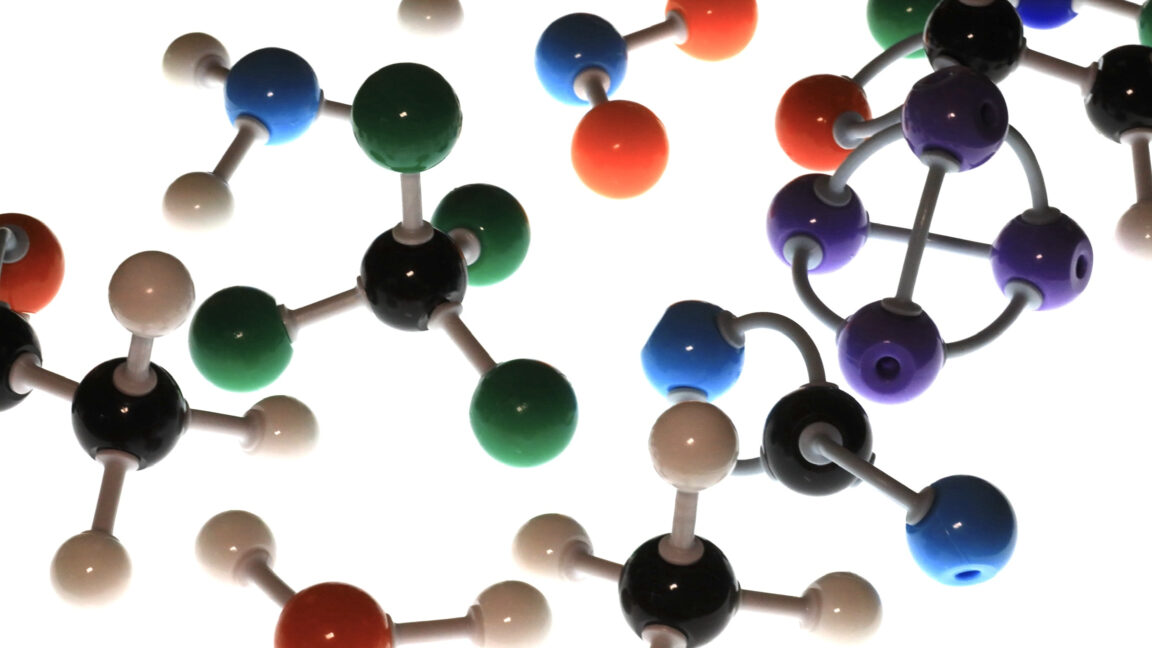
Researchers optimize simulations of molecules on quantum computers
arstechnica.com
The need for speed Researchers optimize simulations of molecules on quantum computers A new approach to simulating the electrons of small molecules like catalysts. John Timmer Jan 24, 2025 10:51 am | 5 Credit: Douglas Sacha Credit: Douglas Sacha Story textSizeSmallStandardLargeWidth *StandardWideLinksStandardOrange* Subscribers only Learn moreOne of the most frequently asked questions about quantum computers is a simple one: When will they be useful?If you talk to people in the field, you'll generally get a response in the form of another question: useful for what? Quantum computing can be applied to a large range of problems, some of them considerably more complex than others. Utility will come for some of the simpler problems first, but further hardware progress is needed before we can begin tackling some of the more complex ones.One that should be easiest to solve involves modeling the behavior of some simple catalysts. The electrons of these catalysts, which are critical for their chemical activity, obey the rules of quantum mechanics, which makes it relatively easy to explore them with a quantum computer.But it's not easy to understand how those simulations will actually work or what limits dictate the hardware we'll need before we can run themgenerally estimated to be around 100 error-corrected qubits. On Wednesday, Nature Physics published a paper that describes the simulation of some aspects of simple catalysts on quantum computers and provides a way to dramatically simplify the calculations. The resulting algorithmic improvements mean that we may not need to wait for error correction to run useful simulations.Spin cityThe behavior of a catalyst depends on its electrons, and their behavior depends on a combination of the orbital they occupy and their spin (which can be up or down). If electrons share an orbital with a partner, they will have opposite spins and essentially cancel each other out. But a lot of chemistry involves unpaired electrons, which have what you might think of as exposed spin. The spins affect the energy levels they can occupy and can interact with unpaired spins elsewhere in the molecule.But spin is a quantum property, with all the complications that ensue from that. And the more unpaired spins a molecule has, the more complicated the interactions among them. The net result is that predicting the spins of a molecule like a catalyst quickly becomes computationally intractable.But since a molecule's spins are a quantum system, it's possible to simulate their behavior on other quantum systems. In fact, a number of approaches to quantum computers, including those involving trapped ions or neutral atoms, store qubits in spins. But fully simulating how the electrons of a molecule interact over time involves a lot of qubits and a long series of the one- and two-qubit gate operations that are central to quantum computations.The new work, performed primarily by researchers at Berkeley and Harvard, describes a method to make a simulation work far more efficiently.Quantum magicThe process begins by using regular computers to simplify a problem to be solved using quantum hardware. This essentially gets rid of some aspects of the problem that aren't particularly relevant to most catalysts, like their behavior under extreme conditions. Instead, it simplifies the description of the system (termed a Hamiltonian) down to focus on the behavior of any unpaired spins when the system is at low energies.The Hamiltonian that describes the spins is then mapped onto a quantum processor, with a cluster of linked qubits devoted to the behavior of each individual spinning electron. This system is generic enough to be mapped onto any quantum hardware, and it can be made to evolve over time, just as the real-world system does.But the researchers found they could get better performance on the quantum side of things by using a quantum computer based on neutral atoms. A typical quantum computing algorithm assumes that all computational operations, termed gates, are done using either an individual qubit or pairs of qubits (these are termed one- or two-qubit gates). But since neutral atoms can be moved around, it's possible to group even more of them so that a small cluster of atoms performs a gate. These multi-qubit gates turn out to allow the specific computations needed here to be performed far more efficiently than they ever could if limited to two-qubit gates.The net result is a much faster operation involving far fewer gates. That's important because errors in quantum hardware increase as a function of both time and the number of operations.The researchers then used this approach to explore a chemical, Mn4O5Ca, that plays a key role in photosynthesis. Using this approach, they showed it's possible to calculate what's called the "spin ladder," or the list of the lowest-energy states the electrons can occupy. The energy differences between these states correspond to the wavelengths of light they can absorb or emit, so this also defines the spectrum of the molecule.Faster, but not quite fast enoughWe're not quite ready to run this system on today's quantum computers, as the error rates are still a bit too high. But because the operations needed to run this sort of algorithm can be done so efficiently, the error rates don't have to come down very much before the system will become viable. The primary determinant of whether it will run into an error is how far down the time dimension you run the simulation, plus the number of measurements of the system you take over that time."The algorithm is especially promising for near-term devices having favorable resource requirements quantified by the number of snapshots (sample complexity) and maximum evolution time (coherence) required for accurate spectral computation," the researchers wrote.But the work also makes a couple of larger points. The first is that quantum computers are fundamentally unlike other forms of computation we've developed. They're capable of running things that look like traditional algorithms, where operations are performed and a result is determined. But they're also quantum systems that are growing in complexity with each new generation of hardware, which makes them great at simulating other quantum systems. And there are a number of hard problems involving quantum systems we'd like to solve.In some ways, we may only be starting to scratch the surface of quantum computers' potential. Up until quite recently, there were a lot of hypotheticals; it now appears we're on the cusp of using one for some potentially useful computations. And that means more people will start thinking about clever ways we can solve problems with themincluding cases like this, where the hardware would be used in ways its designers might not have even considered.Nature Physics, 2025. DOI: 10.1038/s41567-024-02738-z (About DOIs).John TimmerSenior Science EditorJohn TimmerSenior Science Editor John is Ars Technica's science editor. He has a Bachelor of Arts in Biochemistry from Columbia University, and a Ph.D. in Molecular and Cell Biology from the University of California, Berkeley. When physically separated from his keyboard, he tends to seek out a bicycle, or a scenic location for communing with his hiking boots. 5 Comments
0 Comentários
·0 Compartilhamentos
·63 Visualizações