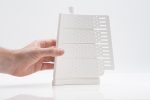
3DPRINTINGINDUSTRY.COM
Ceramic 3D Printing’s Path to Industrialisation with HRL Labs, Lithoz and NIST: 2025 AMUG Conference
Additive manufacturing for functional ceramics continues to push the limits of material science and process engineering, particularly at the intersection of microelectronics, optics, and advanced packaging. At the 2025 AMUG Conference, experts from HRL Laboratories, Lithoz, and the National Institute of Standards and Technology (NIST) dissected the underlying challenges holding back broader adoption of 3D printing with ceramics.
Insights into functional 3D printed ceramics
Photopolymer chemistry is a balancing actOptical distortion from high-refractive fillers needs to be understood.Compensating for shrinkage and porosity to achieve small features or electrical performance is important.Debinding is an often-hidden bottleneck.Process iteration is intensive and material-specific.Vast market opportunity for those who master ceramic 3D printing
Opening the afternoon session, Russell Maier, who leads the ceramics AM programme at NIST, underscored the critical role of feedstock rheology in shaping viable ceramic parts. He warned that differences in equipment and measurement techniques can yield inconsistent rheological data, making standardisation an urgent concern for industry players. “You can measure the yield stress on one instrument and get a value that’s two to three times different than on another,” Maier said. “That’s a huge variability when it comes to deciding if a feedstock is even printable.”
Yield stress is especially important for shaping electroactive ceramics, where high solids loading in slurries must be carefully controlled to ensure structural integrity post-printing. Traditionally, dense ceramics are formed via tape casting and gel casting, where binders and solvents are removed to leave packed ceramic particles. These processes are now being reimagined in additive methods, but still require deep domain expertise. “Even if you become an expert in ceramic AM, you still have to know how to fire and densify it properly. You still need to be a ceramic engineer,” Maier emphasised.
He also pointed to the market opportunity for AM in multi-layer ceramic capacitors (MLCCs), which are ubiquitous in smartphones, automobiles, and industrial equipment. According to Maier, more than 1,000 MLCCs are used in a modern smartphone, and tens of thousands in a single truck. The MLCC market alone is expected to hit $16 billion this year, yet additive methods are just beginning to penetrate this space.
Shawn Allan of Lithoz America provided a closer look at lithography-based ceramic manufacturing (LCM), a DLP-driven printing method that enables the high-resolution fabrication of functional ceramic parts. “We’re essentially replacing the forming step of ceramic manufacturing with 3D printing. Everything else, debinding, sintering, follows traditional processes,” Allan explained.
He presented 3D printed parts made from materials such as yttria-stabilised zirconia, alumina, and transparent ceramics like yttrium aluminium garnet (YAG), which are relevant in optics and laser applications. Notably, LCM allows micron-level feature resolution and tolerances tight enough for components like surgical instruments or dielectric resonators used in 5G and satellite communications.
Allan’s team has worked with federally funded research centers and manufacturers on piezoelectric ceramics, such as PZT, optimizing slurry formulations to match traditional densities and dielectric coefficients. They’ve printed Gaussian transducer arrays and negative Poisson ratio lattices for potential use in sonar, underwater communications, and directional acoustic sensors.
“Eventually, we were able to manufacture a transducer based entirely on a computationally designed lattice. That kind of structure would be extremely difficult to fabricate using conventional methods,” Allan said.
Functional ceramic development is now extending into multi-material printing, including co-sintered systems that combine ceramics with metals like copper or stainless steel. This raises challenges in matching thermal expansion and densification profiles during sintering. According to Allan, future success will hinge on leveraging known, industry-validated materials from traditional processes, “not developing something new, but taking advantage of what already works.”
The path forward is clear: greater industry collaboration on feedstock standards and expanded use of AM for complex, functional ceramics that traditional techniques cannot match in terms of geometry or integration potential.
HRL Pushes Boundaries of Curved Microelectronics with Preceramic 3D Printing and Metal Infiltration
At HRL Laboratories, a cross-functional programme bridging ceramic materials and semiconductor engineering has yielded a functional, curved electronic interposer with high-resolution, high-aspect-ratio electrical vias, manufactured via additive methods. The breakthrough aims to meet the growing demand for compact, high-performance imaging systems and next-generation curved sensor arrays.
Kayleigh Porter, a ceramics specialist at HRL, outlined the lab’s efforts to print complex three-dimensional via arrays with preceramic polymers, using lithography-based 3D printing and a proprietary metal infiltration process. These vias form electrical connections between curved sensor surfaces and planar readout electronics, an architecture that traditional planar microelectronics cannot address.
“Standard microelectronic vias are limited to vertical or horizontal paths, and every directional change introduces signal loss,” Porter said. “With 3D printing, we can design curved or angled vias, embed passive elements, and even coolant channels directly into the part.”
The team used a photosensitive preceramic polymer, a siloxane functionalised with silicon in the backbone, which forms a silicon oxycarbide matrix after pyrolysis. Unlike conventional binders that burn off, this binder becomes part of the ceramic structure, improving material stability and opening up possibilities for functional filler materials.
One of the main goals was to preserve the resolution of a 2 million-pixel curved detector. To achieve this, HRL employed a 2K DLP projection system with a two-micron pixel pitch and tailored the resin’s cure characteristics using photoinitiator blends and kinetic modelling. Maintaining image fidelity across a non-planar geometry required deactivating certain pixels to preserve uniform pitch on the curved surface, essential for optical accuracy.
Filling the tiny vias, some just 10 microns in diameter, posed a metallurgical challenge. Standard electroplating techniques were unsuitable for high-aspect-ratio and curved geometries. HRL developed a copper-indium alloy with trace amounts of titanium to facilitate capillary-driven melt infiltration, achieving a 98% fill rate across a one-millimetre-thick interposer section.
“For the vias to function electrically, they must be continuous from top to bottom,” Porter said. “Incomplete fill leads to dead pixels, so we validated via continuity through imaging and resistance measurements. The successful parts showed strong signal transmission across the entire stack.”
Material compatibility was also a critical consideration. The coefficient of thermal expansion (CTE) needed to match that of the semiconductor substrates, typically silicon or gallium arsenide. Particle additives such as alumina and mullite were screened for shrinkage control and CTE tuning, and para-silica particles were used to minimise refractive distortion during curing.
The interposer’s final metal network was polished to expose a clean metallised interface, then integrated with a thinned gallium arsenide detector chip via spike bonding and fan-out methods. A full electrical test confirmed signal integrity, validating the concept for scaled applications.
While still in the prototype stage, the technology suggests a viable path to compact, high-performance imaging electronics, particularly where conformal packaging and optical curvature offer design advantages. The additive process also allows HRL to rapidly iterate sensor geometries without long lead times or retooling. “You just redesign the part and print it. That’s a huge benefit,” Porter said.
Industry Pushes for Precision in Ceramic Additive Manufacturing as Resolution, Chemistry, and Debinding Remain Obstacles
Russell Maier of the National Institute of Standards and Technology highlighted the material sensitivity required to access the lucrative MLCC market. “You have to be worried about trace concentrations of sodium, titanium—lightweight transition metals can ruin electrical properties,” he warned. Even residual carbon from photoresins, he said, can pose risks when sintered into a dielectric material.
In photopolymer-based ceramic AM, the resin’s optical behaviour and chemical load must be tightly controlled. HRL’s Kayleigh Porter described the complex trial-and-error process of photoinitiator tuning. “We had to mix four or five different resins to really dial in what would work. It’s about pulling multiple levers at once (absorbers, initiators, reflections) and that gets tricky fast,” she explained.
Reflection from high-refractive-index particles also proved problematic. Alumina, a common ceramic filler, scattered light during exposure, limiting HRL’s via resolution to 80 microns. In contrast, Porter noted, using para-silica matched to the matrix index enabled 10-micron feature fidelity. “The trade-off is less mechanical customisation, but you get the resolution,” she said.
Shrinkage, another perennial issue in ceramics, was discussed across multiple speakers. Lithoz America’s Shawn Allan acknowledged the need for compensation, especially in high-resolution prints. “As features approach the pixel or layer size, you can’t rely on the STL anymore. You have to print, measure, and adjust,” he said. Allan also pointed out the challenges with partial densification: “The dielectric constant is coupled with porosity. If there’s trapped gas or incomplete sintering, the value drops.”
Debinding emerged as a critical step with high stakes. “The print might take hours, but the debinding process takes a week or more,” Maier said. “It’s a black box. You go too fast, and you’ve just destroyed a week of work.” NIST typically errs on the side of caution. Gradual burnout helps preserve part geometry, especially where thick and thin sections coexist in the same structure.
Porter noted that HRL’s use of active binders that convert to ceramic reduced porosity but introduced a different issue. “When we load over 25% filler, the matrix shrinks while the particles don’t. That creates internal stress, tension between particles, which can lead to cracking,” she said. HRL has previously published findings on this phenomenon, underscoring the importance of matrix-particle balance.
As ceramic additive manufacturing moves closer to high-value applications, success hinges on managing micro-scale process variability and chemistry with extreme precision. Whether it’s controlling trace contaminants, compensating for shrinkage in vias, or ensuring debinding doesn’t undo a week’s worth of work, the field demands interdisciplinary expertise and deep iteration.
What 3D printing trends should you watch out for in 2025?
How is the future of 3D printing shaping up?
To stay up to date with the latest 3D printing news, don’t forget to subscribe to the 3D Printing Industry newsletter or follow us on Twitter, or like our page on Facebook.
While you’re here, why not subscribe to our YouTube channel? Featuring discussion, debriefs, video shorts, and webinar replays.
Featured image shows a 3D printed ceramic casting core produced on the S320. Photo via Lithoz.
0 Comments
0 Shares
37 Views