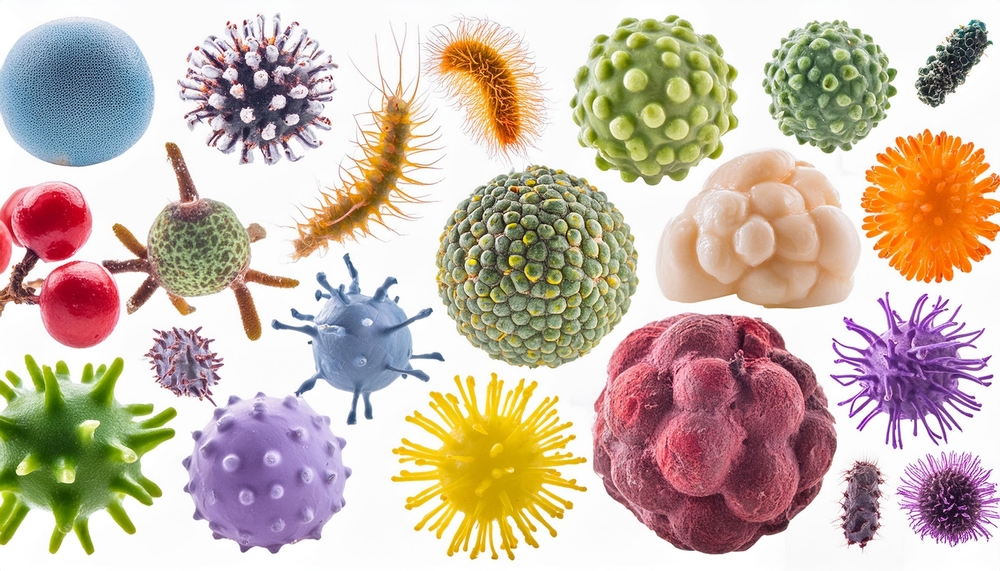
WWW.DISCOVERMAGAZINE.COM
Microbes Can Colonize Space, Produce Drugs, and Create Energy
After so many years of learning how microbes work, researchers are now digitally recreating their inner workings to tackle challenges ranging from climate change to space colonization.In my work as a computational biologist, I research ways to get microbes to produce more useful chemicals, such as fuels and bioplastics, that can be used in the energy, agricultural, or pharmaceutical industries. Traditionally, researchers have to conduct several trial-and-error experiments on Petri dishes in order to determine the optimal conditions microbes need to produce high amounts of chemicals.Instead, I am able to simulate these experiments all from behind a computer screen through digital blueprints that replicate the inside of microbes. Called genome-scale metabolic models, or GEMs, these virtual labs significantly reduce the time and cost required to figure out what researchers need to do to get what theyre looking for. With GEMs, researchers cannot only explore the complex network of metabolic pathways that allow living organisms to function but also tweak, test, and predict how microbes would behave in different environments, including on other planets.As GEM technology continues to evolve, I believe these models will play an increasingly important role in shaping the future of biotechnology, medicine, and space exploration.What Are Genome-Scale Metabolic Models?Genome-scale metabolic models are digital maps of all the known chemical reactions that occur in cells that is, the cells metabolism. These reactions are crucial for converting food into energy, building cellular structures, and detoxifying harmful substances.To create a GEM, I begin by analyzing an organisms genome, which contains the genetic instructions cells use to produce proteins. A type of protein coded in the genome called enzymes are the workhorses of metabolism they facilitate the conversion of nutrients into energy and building blocks for cells.By linking the genes that encode enzymes to the chemical reactions they help make happen, I can build a comprehensive model that maps out the connections between genes, reaction,s and metabolites. (Credit: Chakazul/Wikimedia Commons)This map shows just a few of the major metabolic pathways in cells.Once I build a GEM, I use some advanced computational simulations to make it work like a live cell or microbe would. One of the most common algorithms researchers use to do these simulations is called a flux balance analysis. This mathematical algorithm analyzes available data about metabolism and then makes predictions on how different chemical reactions and metabolites would act under specific conditions.This makes GEMs particularly useful for understanding how organisms respond to genetic changes and environmental stresses. For example, I can use this method to predict how an organism will react when a specific gene is knocked out. I could also use it to predict how it might adapt to the presence of different chemicals in its environment or a lack of food.Solving Energy and Climate ChallengesMost of the chemicals used in agriculture, pharmaceuticals, and fuels are obtained from fossil fuels. However, fossil fuels are a limited resource and significantly contributeto climate change.Instead of extracting energy from fossil fuels, my team at the Great Lakes Bioenergy Research Center of the University of Wisconsin-Madison focuses on developing sustainable biofuels and bioproducts from plant waste. This includes cornstalk after the ears are harvested and nonedible plants such as grass and algae. We study which crop wastes can be used for bioenergy, how to use microbes to convert them into energy, and ways to sustainably manage the land on which those crops are grown.I am building a genome-scale metabolic model for Novosphingobium aromaticivorans, a species of bacteria that can convert very complex chemicals in plant waste to chemicals that are valuable to people, such as those used to make bioplastics, pharmaceuticals, and fuels. With a clearer understanding of this conversion process, I can improve the model to more accurately simulate the conditions needed to synthesize greater amounts of these chemicals.Researchers can then replicate these conditions in real life to generate materials that are cheaper and more accessible than those made from fossil fuels.Bioinformatics analyzes biological data to answer questions about living organisms.Extreme Microbes and space colonizationThere are microbes on Earth that can survive in extremely harsh environments. For example, Chromohalobacter canadensis can live in extremely salty conditions. Similarly, Alicyclobacillus tolerans can thrive in very acidic environments.Since other planets typically have similarly harsh climates, these microbes may not only be able to thrive and reproduce on these planets but could potentially change the environment so humans can live there as well.Combining GEMs with machine learning, I saw that C. canadensis and A. tolerans can undergo chemical changes that help them survive in extreme conditions. They have special proteins in their cell walls that work with enzymes to balance the chemicals in their internal environment with the chemicals in their external environment.With GEMs, scientists can simulate the environments of other planets to study how microbes survive without necessarily needing to go to those planets themselves.The Future of GEMsEvery day, researchers are generating large amounts of data about microbial metabolism. As GEM technology advances, it opens the door to exciting new possibilities in medicine, energy, space, and other areas.Synthetic biologists can use GEMs to design entirely new organisms or metabolic pathways from scratch. This field could advance biomanufacturing by enabling the creation of organisms that efficiently produce new materials, drugs, or even food.Whole human body GEMs can also serve as an atlas for the metabolics of complex diseases. They can help map how the chemical environment of the body changes with obesity or diabetes.Whether producing biofuels or engineering new organisms, GEMs provide a powerful tool for basic research and industrial applications. As computational biology and GEMs advance, these technologies will continue to transform how scientists understand and manipulate the metabolisms of living organisms.Blaise Manga Enuh is a Postdoctoral Research Associate in Microbial Genomics and Systems Biology at the University of Wisconsin-Madison. This article is republished from The Conversation under a Creative Commons license. Read the original article.
0 Комментарии
0 Поделились
55 Просмотры